Late crystallization of the lunar magma ocean and the origin of KREEP
Welsch, François
Promotor(s) :
Charlier, Bernard
Date of defense : 5-Sep-2019 • Permalink : http://hdl.handle.net/2268.2/7441
Details
Title : | Late crystallization of the lunar magma ocean and the origin of KREEP |
Translated title : | [fr] Cristallisation tardive de l'océan magmatique lunaire et origine des KREEP |
Author : | Welsch, François ![]() |
Date of defense : | 5-Sep-2019 |
Advisor(s) : | Charlier, Bernard ![]() |
Committee's member(s) : | Namur, Olivier
Vander Auwera, Jacqueline ![]() Debaille, Vinciane |
Language : | English |
Number of pages : | 68 |
Keywords : | [en] Experimental petrology, [en] Liquid immiscibility [en] urKREEP [en] Phase equilibria |
Discipline(s) : | Physical, chemical, mathematical & earth Sciences > Earth sciences & physical geography |
Target public : | Researchers Professionals of domain Student General public Other |
Institution(s) : | Université de Liège, Liège, Belgique |
Degree: | Master en sciences géologiques, à finalité approfondie |
Faculty: | Master thesis of the Faculté des Sciences |
Abstract
[en] After 99 % of the LMO crystallization, a residual liquid enriched in K, REE and P known as the urKREEP remained. In its latest stages, the urKREEP might have undergone silicate liquid immiscibility producing a ferrobasaltic melt forming the REEP-fraction (enriched in REE, Fe, Ca and Ti) and a granitic melt forming the K-fraction (enriched in Si, Na, K and Al). This liquid separation might be a significant process in the granite formation and phosphates by metasomatism of the ferrobasaltic melt during its ascent. This study aimed at understanding the residual liquid evolution of the magma ocean and had a special interest on the formation of large immiscible droplets. The mineral phases, textures and the influence of parameters (starting temperature, equilibrium time) were also studied. We used Pt-loops in the controlled-atmosphere furnace over 1140-980 °C under reducing conditions (Iron-Wustïte buffer) to produce melt compositions reproducing an evolution by fractional crystallization. First, the Pt-loops were saturated in iron to remove any iron loss from the powders. These powders were stuck on a Pt-loop and placed into the controlled-atmosphere furnace at the starting temperature. A cooling rate of 1 °C/h were used and once the target temperature was reached, maintained for 72h before quenching. Mineral phases were analysed by using the electron microprobe at the university of Aachen. Added to these results, data of isothermal experiments were used to compare immiscible droplets and better constraint the liquid evolution until the onset of immiscibility.
Most experimental products contain olivine, pyroxene, plagioclase, a silica phase (trydimite) and ilmenite. Some show Fe metal, whitlockite, ulvöspinel and two melts. The two-liquid field was reached at 1000 °C where Si-rich granitic melt droplets are formed. Cooling experiments resulted in small size immiscible droplets, while those from isothermal experiments were larger.
During cooling of the LMO residuum, the residual liquid evolved towards a Fenner trend by being enriched in Fe, K, P, Ca and a depletion in Al, Ti enriched until ilmenite saturation and then get depleted. At 1000 °C, the residual liquid entered the two-liquid field producing a granitic melt. Phosphate saturation was only reached in bulk Moon composition enriched in phosphorous. This lack of phosphates prior to immiscibility suggested that they might be formed by the ferrobasaltic melt. As cooling proceeds, ilmenite and fayalite crystallized from the ferrobasaltic melt promoting the rise of this one by metasomatism. However, when metasomatism occurs, a mineral phase is formed beneath the melt: a phosphate. The ferrobasaltic melt being Fe-rich, the first crystallizing phosphate might be a whitlockite and in the late stages of the ferrobasaltic melt, apatite as the melt becomes enriched in halogens.
Almost all experimental products showed a porphyritic texture prior to silicate liquid immiscibility (with only one intergranular texture) and immiscible texture once immiscibility is reached. The little diversity of these textures suggested that using a sufficiently slow cooling rate and decreasing the starting temperature lead to similar textures. Moreover, the equilibrium time seemed to have an impact on the size of immiscible droplets: long equilibrium time produced large immiscible droplets and a mature immiscible texture while shorter ones produced small droplets and an immature texture.
The origin of lunar granite has two main origins: 1) Production of small volume of granite by silicate liquid immiscibility in depth (their low viscosity preventing them from migrating). 2) Basaltic underplating producing large volume of silicic magma and possibly the cause of silicic volcanism.
File(s)
Document(s)
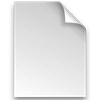

Description:
Size: 2.24 MB
Format: Adobe PDF
Annexe(s)
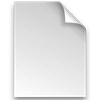

Description:
Size: 172.84 kB
Format: Adobe PDF
Cite this master thesis
The University of Liège does not guarantee the scientific quality of these students' works or the accuracy of all the information they contain.